All the living organism needs energy to perform their different normal metabolic functions. Food is the source of the power of the living body. Chlorophyll-containing plants produce foods by using solar energy. This particular function for creating their food is known as photosynthesis. French physiologist Henri Dutrochet (1837) first showed that the green parts of a plant play an important role in photosynthesis.
American botanist Charles Reid Barnes coined the term photosynthesis in 1893. The green plants synthesize massive amounts of food materials with the help of light energy. During photosynthesis, carbohydrates are produced, which constitute the necessary raw materials that contain all types of organic compounds needed for the entire living world.
Photosynthesis is the process by which the chlorophyll-containing plants and some other organisms use light energy to produce glucose (C6H12O6) and oxygen with the presence of water (H2O) and carbon dioxide (CO2). In this case, light energy is converted into chemical energy and is stored as carbohydrates. Here, glucose can be converted into pyruvate, which releases ATP by cellular respiration. The following equation summarizes photosynthesis.


Organs for Photosynthesis
The mechanism of photosynthesis occurs within the cell organelle, chloroplastid, which is present in the green plants. Chloroplast comprises of the double-membrane body, which acts as a selective carrier to the movement of cellular metabolism in or out of the chloroplast. The membrane is a unit membrane and is approximately 50-70Å thick. The proteinaceous liquid matrix is called stroma. Thylakoids are the membrane-bound sac-like structures embedded within the stroma. Thylakoids are arranged in stacks that constitute the grana and which are further interconnected by membrane-bound channel-like structures, called stroma lamellae. The inner surface of the thylakoid bears distinct morphological structures, which are called quantasomes. These quantasomes bear many chlorophyll molecules and accessory proteins that are capable of capturing and converting solar energy into chemical energy, thus acting as a photosynthetic unit. In the case of photosynthetic bacteria, the necessary pigments, enzymes are located in vacuolar membrane enveloped structure, called chromatophores, Light reaction occurs within the grana while dark reaction occurs within the ground substance, the stroma of the chloroplast.
Site of Photosynthesis
Photosynthesis occurs in chlorophyll-containing leaves of plants. Chloroplast containing chlorophyll is present in abundance in the mesophyll tissue of the leaves. Cells of the mesophyll tissue are the leading site for photosynthesis. But the green part of a plant body can able to do photosynthesis. Photosynthesis also occurs in the green part of a growing stem such as Opuntia stricta, Lagenaria. Photosynthesis also takes place in the thalamus of flower (it is a modified stem), in the roots, in sepals, in the roots of orchids due to the presence of spongy tissue( it is also known as velamen) and even in unicellular organisms like Euglena, Chlamydomonas, etc.
Higher plants carry out photosynthesis within the membrane-bound structure, chloroplast. Several hundred chlorophyll molecules and a small number of accessory pigment molecules such as pili proteins, carotenoids, etc. functions as independent photosynthetic units and capture light energy.
These photosynthetic units are present in some small particles in the membranous granum disc, which are called quantasomes. The second stage photosynthesis occurs within the stroma of the chloroplast. In the blue-green algae and certain bacteria, photosynthesis occurs in the chromatophores. In aquatic plants, dissolved CO2 and H2O diffuse throughout the body surface.
Adaptation of Plant`s Leaves for Photosynthesis
- Generally, the leaves have a flattened shape, and they provide a large surface area to light.
- The midrib and veins of leaves are surrounded by tissues that impart strength to the leaf and maintain the original shape.
- A thick cuticle is present on the upper surface and a thin cuticle on the lower surface to prevent excessive water loss due to this large surface area.
- Stomatal openings are present in the leaves through which the exchange of gasses takes place with the atmosphere.
- Veins of leaves create network and supply of water to photosynthetic cells, and quick disposal of photosynthetic products occur.
- Intercellular air spaces allow easy diffusion of gases throughout the whole leaf.
Components of Photosynthesis
The components of photosynthesis are:
- Carbon dioxide(CO2)
- Water (H2O)
- Chlorophyll and
- Sunlight
Carbon dioxide (CO2): Terrestrial plants get gaseous CO2 through the stomata of the leaves from the atmosphere, which later on enters the mesophyll tissue of leaves. CO2 can also enter a plant body through lenticel and cuticle. CO2 at first dissolves with the cuticular substances and enters the leaf through the diffusion process.
In this case, a chemical reaction occurs between water and CO2 in the intercellular spaces, and carbonic acid is formed, which is harmful to protoplasm. That is why it is converted to form bicarbonate (HCO3−). This bicarbonate plays an essential role in the pH status of the cell in all organisms. The cell wall of the nearby cells of the intercellular space receives this bicarbonate by a diffusion process, enters the cell, and then into the chloroplast. Most of the gaseous CO2 is needed for photosynthesis, which is obtained from the atmosphere by the plants. Further, the CO2 is obtained by respiration, and other metabolic reactions may also act as the source of CO2 for photosynthesis. During the day time, the stomatal pores generally remain open through which gaseous CO2 enters the leaves. In the case of submerged aquatic plants, CO2 enters the plant body by the diffusion process through the plant surface.
The amount of CO2 in the atmosphere is about 0.03-0.04 %. In the mesophyll tissue of the leaf, water is present. This water, along with CO2 forms carbonic acid (H2CO3). In the presence of sunlight, the carbonic acid is again formed CO2 and H2O. This CO2 is used up in the photosynthetic mechanism.
Water (H2O): Generally, plants receive water by the process of osmosis from the soil through the unicellular root hairs. This absorbed water reaches xylem vessels, and later on, it is transported by several phenomenons to the intercellular space of the mesophyll tissue of leaves. From the cell wall, water is transported to the cell and then to the chloroplast through the process of diffusion. Plants get their necessary amount of water from the soil. They also get water during respiration and other metabolic functions. Plants require about 1% of the total amount of water during photosynthesis.
The solar energy is transformed into chemical energy by the chlorophyll molecules. The electrons of chlorophyll molecules get excited by the photons and water molecule breaks up into H+ ions, and OH– ion utilizing light-activated chlorophyll. From OH– ions, oxygen is formed, later on, which is also liberated out. The process of splitting up of water utilizing light-activated chlorophyll is called photolysis. Generally, photolysis is a chemical reaction which is also known as photodecomposition or photodissociation.
Nicotinamide Adenine Dinucleotide Phosphate (NADP+) is converted to NADPH by receiving the H+ ion from H2O .
Chlorophyll: Chlorophyll tissue is present in the mesophyll tissue of leaves. Photosynthesis occurs within the chloroplasts. Plants may contain two types of pigments: green colored chlorophyll and yellowish red colored chlorophyll. Chlorophylls are of two types; chlorophyll-a and chlorophyll-b, of which chlorophyll-a is more abundance. Carotenoids are of two kinds: orange-colored carotene and yellow-colored xanthophyll. Apart from these, chlorophyll c, d, and e are also present. Other photosynthetic pigments are blue phycocyanin and red phycoerythrin (PE). The chemical formula of different photosynthetic pigments is given below:
- Chlorophyll a-C55H72N4Mg
- Chlorophyll b-C55H70O6N4Mg
- Carotene-C40H56
- Xanthophyll-C40H56O2
- Phycocyanin-C34H44O8N4
- Phycoerythrin-C34H46O8N4
Chlorophyll atoms are arranged in a circle with the help of four pyrrole rings (C4H5N), with Mg atom at the center. Along with the pyrrole ring, phytol (C20H40O) is attached (phytol is a type of alcohol).
Light: Plants require energy to perform the mechanism of photosynthesis, which is obtained from solar radiation.This physiological function of plants can be performed by obtaining sufficient energy from the external sources. Sometimes enough light energy from powerful bulbs or other means may also act as the source of light. That is why it is easy to understand why photosynthesis does not occur at night. The answer is stomatal pores are closed at night. The colored pigments absorb the red, blue, and violet rays of sunlight. Of all the photosynthetic pigments, only a particular form of chlorophyll-a, which is termed P-700 can utilize light energy directly during the photosynthesis. So this is called primary pigment, and other pigments are called accessory pigments. Of the solar radiation, about 5% pass out through the leaf. 13% gets reflected, and 82% is absorbed by the leaf. Again 4% of the 82% that is absorbed by the leaf, and the chlorophyll further absorbs it. Light is composed of high energized invisible particles, called quanta or photons, which are capable of activating the chlorophyll molecules.
Mechanism of Photosynthesis
Photosynthesis is a complex mechanism. The process is concerned with the assimilation of carbon in the presence of light; hence, it is also called as carbon assimilation. During the process, CO2 is reduced up to the level of carbohydrates. The process is also involved in the liberation of oxygen as a byproduct. The overall equation for the process is:


The formation of glucose in the photosynthesis involves a series of reactions which occur in the light as well as darkness constituting the light phase or the light-dependent reactions and dark phase or light-independent reactions, respectively.
Photosynthesis occurs by two stages, which were stated by scientist Frederick Frost Blackman in 1905. In this case, light-dependent reactions are held in the grana of chloroplast in the presence of light, while the light-independent reactions are held in the stroma of chloroplast in the absence of light. The light-dependent reactions produce ATP and NADPH2, and the light-independent reactions produce carbohydrates.
Light Phase or the light-dependent Reactions
The light-dependent reactions are also known as photo-reduction. It is the first stage of photosynthesis. It is a process by which plants capture and store energy from sunlight. The main function of these reactions is to provide a source of ATP and reduced NADP, which are used to reduce CO2 in the light-independent reaction. At first reaction, the chloroplast absorbs the photons of light and transfer them ATP as chemical energy. In another reaction, water is split into an electron (2e–), proton (H+), and O2 in the presence of Mg++ and Cl–. This splitting of water by light is called photolysis. The hydrogen then reduces NADP into NADPH2. The following equation summarizes the light reaction.


The phosphorylation of ADP to form ATP using the energy of sunlight is called photophosphorylation. Only two sources of energy are available for living organisms: sunlight and reduction-oxidation reactions.
Light Trapping System: During the light-dependent phase, light is trapped by the photosynthetic pigments which are present in the quantasomes of the grana and thylakoids. These pigments are organized into two pigment systems called pigment system-I (PS-I) and pigment system-II (PS-II). In this case, PS-I is composed of chlorophyll-a 700(P700), chlorophyll-a 683, and carotenoids, while PS-II is composed of chlorophyll-a 680(P680), chlorophyll-a 670, and carotenoids.
In both PS-I and PS-II systems, all types of pigment molecules aid in trapping light energy. On the other hand, all other molecules shift their energy to the reaction center for the photochemical reactions during photosynthesis. During the light reactions, ATP formation takes place through two types of phosphorylation reactions. These are Non-cyclic photophosphorylation and cyclic photophosphorylation. In this case, the non-cyclic process involves both PS-I and PS-II, while the cyclic process involves only PS-I.
Non-cyclic Photophosphorylation
Non-cyclic photophosphorylation is the process where ATP is formed from ADP and H3PO4 by using energy from the excited electrons provided by PS-II (photosystem-II). In this case, the transfer of electrons occurs in a unidirectional or in a non-cyclic manner. It is called non-cyclic photophosphorylation because the lost electrons by P680 of PS-II are taken up by P700 of PS-I and which are not overturn back to P680.
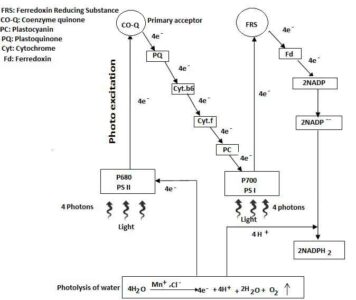
Non-cyclic Photophosphorylation
Some important features of non-cyclic photophosphorylation are:
- It is a photochemical reaction and is the major pathway of the light reaction of photosynthesis.
- It occurs in the grana of chloroplasts, where it requires the participation of both PS-I and PS-II.
- In this case, the transfer of electrons through the ETS (electron transfer system) is non-cyclic.
The mechanisms of non-cyclic photophosphorylation are described in the following headlines:
Photoexcitation of PS-I: The chlorophyll-a (P 700) of PS-I is activated on receiving photons of light, and thus it expels electrons. As a result of this loss, it becomes ionized chl-a+. The electrons from PS-I are first accepted by ferredoxin reducing substances (FRS) and are transferred to coenzyme NADP via ferredoxin (Fd). NADP keeps hold of the electrons and is thus reduced.
Photoexcitation of PS-II: Similarly, on receiving photons of light, the chlorophyll-a (680) of PS-II also gets activated and expels electrons. As a result, it becomes ionized chl-a+. The high energy containing electrons from PS-II is first accepted by an electron acceptor PQ (plastoquinone) and are then transferred along with the electron transport system (ETS) consisting of plastoquinone(PQ), cytochrome B6(Cyt B6), cytochrome f(Cyt f) and plastocyanin (PC). Finally, the electrons are accepted by the ionized chl-a+ of PS I. In the way, ionized PS I comes back to the ground state and can again participate in the process.
Photolysis of water: The ionized chlorophyll-a+ of PS II is brought back to the ground state with the help of the electrons made available through the photolysis of water.


Formation of NADPH2 and Liberation of O2: During the photolysis of water, O2 is released as a byproduct. The 2 NADP receive 4e– from PS I and 4H+ from the photolysis of water to form reduced coenzyme NADPH2.


Non-cyclic Electron Transfer: In this process, the movement of electrons is considered to be non-cyclic or unidirectional. This is because; the final electron acceptor is different from the initial electron donor. This process is also called the Z scheme electron transport due to its zig-zag path of the electrons.
ATP Formation: During the non-cyclic transfer of energy-rich electrons through the Electron Transport System (ETS) from PS II to PS I, energy from the electron is released. This is used for the formation of ATP from ADP and H3PO4. This is called non-cyclic photophosphorylation.
End Product of Non-cyclic Process and the Assimilatory Power: The end products are ATP, NADPH2, and O2. Of these, O2 is liberated from the green plants. ATP and NADPH2 are used in the following dark reactions (phase II) for carbon assimilation. In this case, the reduction of CO2 is occurred to form carbohydrates. ATP and NADPH2 of light reactions are known as assimilatory power.
Cyclic Photophosphorylation
Cyclic photophosphorylation is the process where ATP is formed from ADP and H3PO4 in the presence of light and chlorophyll-a with the help of the cyclic transfer of electrons. Some essential features of cyclic photophosphorylation are:
- It is a photochemical reaction that depends on light energy.
- It occurs in the grana of chloroplasts, which require the participation of PS I only.
- In this process, the movement of electrons is a cyclic way, and it involves only ATP formation.
Cyclic photophosphorylation involves the following steps:
Photoexcitation of PS I: The reaction center of PS I (chl-a, i.e. p 700) is activated on receiving light energy in the form of photons. In this case, the chl-a of PS I expels an electron and becomes ionized chl-a+.
Cyclic Electron Transfer: The energy-rich electrons from the PS I are first accepted by ferredoxin reducing substances (FRS) and transferred to ferredoxin(Fd). From Fd, the electrons are transferred to cytochrome B-6, cytochrome f, and then to PC(Plastocyanin). From PC, the particles are finally accepted by the same ionized chl-a+ of PS I. On receiving the lost electrons, the chlorophyll-a+ comes back to the ground state and can again participate in the process. In this process, the initial donor and the final acceptor of electrons are the same chlorophyll-a of PS I. Hence, the movement of electrons is cyclic.
Synthesis of ATP: During the cyclic transfer of the energy-rich electrons, there is a loss of electron energy at each transfer. It is used at once such transfer for the formation of ATP from ADP and H3PO4. This process is known as cyclic photophosphorylation.
Production of Light Reaction: The overall light reaction consists of the non-cyclic and the cyclic photophosphorylation reactions. The products of the light reaction are ATP, NADPH2, and O2. In this case, O2 is liberated from the green plants. ATP and NADPH2 are used in the dark reaction or dark phase for the reduction of CO2 to form carbohydrates (carbon assimilation). Hence, these products of the total light phase (ATP and NADPH2) are known as assimilator power.
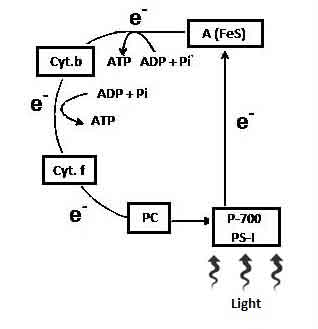
Cyclic Photophosphorylation
The Light-independent Reaction or Dark Reaction
Frederick Frost Blackman first established the dark reaction or light-independent reactions in 1905. For this reason, this reaction is also known as Blackmann Reactions. Thermo-chemical reduction of CO2 involves in this reaction to form carbohydrates. This reaction can take place with or without light. Hence, it is also called light-independent reactions. It occurs in the stroma of the chloroplast, and it plays a vital role in producing organic compounds (i,e. carbohydrates) with the help of ATP and NADPH2. The light-independent reactions have the following two well-recognized paths:
- Calvin Cycle or Calvin Bessham Pathway or C3 Cycle
- Hatch and Slack Pathway or C4 Cycle
Calvin Cycle or Calvin Bessham Pathway or C3 Cycle:
Melvin Calvin, James Bassham, and Andrew Benson discovered this cycle in 1947 using the radioactive isotope, carbon-14(14C). For this reason, this cycle is also called the Calvin cycle or Calvin-Benson-Bassham (CBB) cycle. In 1961, Melvin Calvin and his colleagues rewarded the Nobel Prize in Medicine and Physiology due to the discovery of this cycle. Three carbon phosphoglyceric acid is the first product component of this cycle. Hence, this cycle is also known as C3 Cycle. More than 95% of plant species are the C3 plants on the earth, which make a three-carbon compound as the first stable product of carbon fixation. The Calvin cycle has the following four main steps:
- Carboxylation
- Phosphorylation
- Synthesis of carbohydrates
- RuBP resynthesis
Carboxylation
It is the first step of the Calvin cycle. To start the process of photosynthesis, natural CO2 from the atmosphere enters into the stroma of the chloroplast and merge with pentose sugar ribulose 1,5 biphosphate (RuBP) to form temporary keto acid. RuBisCo (Ribulose-1, 5-bisphosphate carboxylase oxygenase) enzyme catalyzes this reaction.
Promptly, the temporary keto acid hydrolyzes with the help of water and produces two molecules of 3-phosphoglyceric acid (3-PGA). This 3-PGA is treated as the first permanent compound of the Calvin cycle.
Phosphorylation
It is the second step of the Calvin cycle. In this step, energy reacts with the chemicals to create simple sugar 3-phosphoglyceraldehyde (3PGAL).
- 3-phosphoglyceric acid (3-PGA) receives one molecule of inorganic phosphate from ATP, which is produced in light reaction and converted into 1,3-biphosphoglyceric acid (1,3-BPGA). The enzyme phosphoglycerate kinase regulates this reaction. Here, one molecule of ADP is released.
- 1,3 bisphosphoglyceric acid reduce by NADPH2 into 3-phosphoglyceraldehyde(3-PGAL) with the help of phosphoglyceraldehyde dehydrogenase enzyme. Here, one molecule of NADP is released.
- 3-phosphoglyceraldehyde (3-PGAL) can transform into dihydroxyacetone phosphate with the help of the isomerase enzyme.
- In each round of the Calvin cycle, six molecules of CO2 and six molecules of water are used to produce 12 molecules of 3-phosphoglyceraldehyde (3-PGAL). This 3-phosphoglyceraldehyde (3-PGAL) is used in two ways, Only two molecules of 3-phosphoglyceraldehyde (3-PGAL) are used in the synthesis of carbohydrates, whereas ten molecules of 3-phosphoglyceraldehyde (3-PGAL) are used in RuBP synthesis.
- Among ten 3-phosphoglyceraldehyde (3-PGAL), five isomerizes into dihydroxyacetone phosphate (DHAP) by triose phosphate isomerase enzyme.
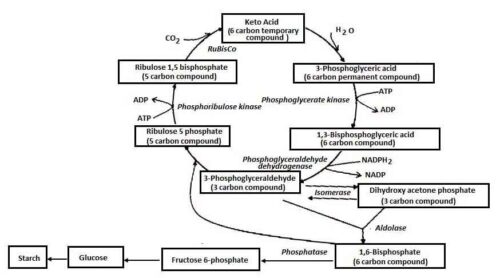
Calvin Cycle
Synthesis of Carbohydrates
It is the third step of the Calvin cycle. In this step, carbohydrates are produced.
- One molecule of 3-phosphoglyceraldehyde(3-PGAL) hydrolyzes with one molecule of dihydroxyacetone phosphate and form one molecule of 1,6 bisphosphate. This reaction is regulated by aldolase enzyme.
- By losing one molecule of phosphate, 1, 6 bisphosphates is transformed into fructose 6-phosphate. This reaction is catalyzed by the phosphatase enzyme. This fructose 6-phosphate is then converted into glucose and starch through a series of successive reactions.
- RuBP resynthesis: It is the fourth and final step of the Calvin cycle. In this final step, energy and sugar act together to form RuBP
- 3-phosphoglyceraldehyde (3-PGAL) and 1,6-bisphosphate combine and form ribulose 5 phosphate through different reactions.
- Ribulose 5 phosphate(C5H11O8P) get one molecule of phosphate from ATP and produce ribulose 1,5 bisphosphate(RuBP) in the presence of phosphoribulase kinase enzyme. This RuBP re-enters in the Calvin cycle and continues the cycle.
Some Facts About Calvin Cycle
- Ribulose diphosphate is the primary acceptor of carbon dioxide (CO2).
- A 3 carbon compound such as phosphoglyceric acid is the first stable product.
- Photorespiration occurs in some plants bearing a type of chloroplast which performs the Calvin cycle.
- Atmospheric oxygen is less used here.
- In this cycle, RuDP is regenerated.
Hatch and Slack Pathway or C4 Cycle
After the fixation of CO2, oxaloacetic acid (4-carbon dicarboxylic acid) is the first stable product in some plants. These plants are known as C4 plants where dark reaction or the C4 pathway occurs. American biologist Hugo Peter Kortschak first noticed this pathway during the photosynthesis of sugarcane leaves in 1957. Marshall Davidson Hatch and C. R. Slack described the details about the C4 pathway in 1966. Hence, it is known as the Hatch and Slack Pathway. For example, in nature, the C4 pathway occurs in maize, sugarcane, some dicots, and monocots plants.
Steps of C4 Cycle
C4 pathway occurs in the two regions of the leaves, such as stroma of the mesophyll cells and stroma of the bundle sheath cells.
Step Occurs in Mesophyll Cells
First Time Fixation of CO2: Atmospheric CO2 combines with three carbon phosphoenol pyruvic acid (C3H5O6P) to form stable product 4 carbon oxaloacetic acid(C4H4O5). Phosphoenol carboxylase enzyme catalyst this reaction.
Oxaloacetic acid (4 carbon compounds) is dehydrogenated by NADPH2 and forms malic acid (C4H6O5) or aspartic acid (C4H7NO4), which is moved to bundle sheath cells. In this case, this reaction is catalyzed by malic dehydrogenase enzymes. At the end of the reaction, a molecule of NADP is released.
Step Occurs in Bundle Sheath Cells
In the presence of decarboxylase enzyme and NADP, malic or aspartic acid undergoes decarboxylation and forms CO2 and pyruvic acid (C3H4O3). This reaction occurs in the chloroplast of the bundle sheath cells. At the end of the reaction, a molecule of NADPH2 is released.
Second Time Fixation of CO2
When decarboxylation of malic acid occurs, CO2 is released, which combines with ribulose 1,5-bisphosphate (RuBP) and forms two molecules of phosphoglyceric acid (C3H7O7P). Further conversion of phosphoglyceric acid occurs and produces sugars.
Decarboxylation of malic or aspartic acid produces pyruvic acid and ships again to the mesophyll cells. With the help of pyruvate kinase enzyme, pyruvic acid is converted into phosphoenol pyruvic acid( C3H5O6P) which again produce available for the C4 pathway.
In the C4 plants, CO2 fixation occurs twice; of which, the first one occurs in the mesophyll cells and the last one in the bundle sheath cells. For this reason, the C4 pathway is also known as a decarboxylation pathway. Besides these, both C4 and C3 pathways occur in C4 plants.
Conditions Necessary for Photosynthesis
The rate of photosynthesis is conditioned by a number of external and internal factors. Light, temperature, CO2, O2, and H2O are the primary external factors, whereas the internal factors are chlorophyl, increasing and decreasing of stomatal aperture, presence of potassium, the structure of the leaf, protoplasm respectively.
Light: Rate of photosynthesis is influenced by intensity, quality, and duration of light. Photolysis of water occurs only in the presence of light. Too much-storing light may even stop the process of photosynthesis. Rete of photosynthesis is directly related to the duration of light.
Temperature: Photosynthesis may occur within the range of temperature between 00C-450C, but optimum temperature ranges from 250C to 350C. With the increase in temperature, the rate of photosynthesis generally increases when other factors are favorable.
Carbon dioxide (CO2): This is one of the essential raw materials used in the process of photosynthesis. Air contains 0.3-0.4% of CO2. An increase in CO2 concentration up to 0.1% approximately increases the rate of photosynthesis. The higher concentration of CO2 is, however, toxic to plants, and it slows down the rate of photosynthesis.
Oxygen (O2): The rate of photosynthesis is decreased with increasing oxygen. This is due to the reason that the enzyme involved in photosynthesis is inactivated under such a condition.
Water (H2O): Water is the raw materials utilized in photosynthesis. The rate of photosynthesis decreases in drought conditions. The effect of water is indirect since deficient water supply induces a reduction in the rate of diffusion of CO2 by closing stomata and causes hydration of protoplasm.
Chlorophyll: It is the green-colored pigment that becomes activated in the presence of light and splits water subsequently. Light energy captured by the plant is converted to the chemical energy of glucose, which is formed as a result of photosynthesis.
Increasing and decreasing of stomatal aperture: Through stomatal pore, the necessary amount of CO2 enters the leaves. Light regulates the opening and closing of stomatal aperture.
Potassium: As a result of photosynthesis glucose is formed. This should be transformed into starch; that is why potassium is necessary.
Structure of leaf: Photosynthesis depends on the structure and proper functioning of the mesophyll tissue, guard cell, etc.
Protoplasm: Protoplasm contains enzymes, coenzymes, cofactors that directly influence the rate of photosynthesis. Proper hydration of protoplasm is also essential for photosynthesis since water influences the chloroplast activity.
Significance of Photosynthesis
Storage of energy: Solar energy is stored in the form of chemical energy in the food, as a result of photosynthesis. This stored energy is used up by the living organisms for performing several physiological functions.
Synthesis of food: Glucose or sugar is produced as a result of photosynthesis, which can be transformed into other carbohydrates, fats, and proteins. These are used in the biosynthesis of other organic compounds. Heterotrophic organisms such as both man and animals are dependent directly or indirectly on the autotrophic green plants.
Energy comes from various foods that are required for the operation of various vital processes.
CO2-O2 Balance: All animals inhale O2 from the atmosphere for respiration and exhale CO2, which is taken in by the green plants during photosynthesis. Thus the stability of CO2 and O2 is maintained in the ecosystem. Again oxygen is released in the atmosphere, which purifies the air.
Photosynthesis and Human civilization: Cellulose present in the cell wall of plants is prepared by the condensation of glucose molecules produced during photosynthesis. This is an important raw material in the textile industry and the paper industry. Wood is a plant product, and coal is a plant fossil. Petroleum and various natural gases are often produced as a result of the decomposition of plant parts. The energy which is stored up in the photosynthetic plants is ultimately released during the combustion of these fuels. In any ecosystem, a green plant represents the essential biotic components as they are the primary producers. Photosynthetic activity of green plants gives rise to industrial products like textile fibers, timber, pulp products, vegetable fats, gums, resins, etc.
Basic commodities for everyday life: Cloth, wooden furniture, rubber goods, plastic, nylon, rayon, paper, etc. are prepared from plants. Cellulose and fiber are the raw materials used in the textile industry. Cellulose is also used in the paper industry.
Oxygen: Oxygen produced as a byproduct of photosynthesis is utilized during combustion and respiration.
Difference between Cyclic and Non-Cyclic Photophosphorylation
Cyclic Phosphorylation |
Non-cyclic Phosphorylation |
---|---|
In this process, water is not required. |
In this process, water is required. |
In this system, oxygen is not produced. |
In this system, oxygen is produced as a byproduct. |
In one cycle path, 2 ATP molecules are formed. |
In one cycle path, 1 ATP molecule is formed. |
Chlorophyll acts as an electron donor and acceptor. |
One electron donor accepts electron from another. |
In this system, NADPH is not synthesized. |
In this system, NADPH is synthesized. |
Electron does not reduce terminal carrier. |
Electron reduces terminal carrier. |
In this case, only Photosystem I is involved. |
In this case, both Photosystem I and II are involved. |
Electrons revert back to Photosystem I |
Electrons from Photosystem I are accepted by NADP. |
Transportation of electrons occurs in a cyclic manner. |
Transportation of electrons occurs in a no-cyclic manner. |
In this case, P700 is the active reaction center. |
In this case, P680 is the active reaction center. |
This system is predominant only in bacteria. |
This system is predominant in all green plants. |
Difference between Light phase and Dark phase of Photosynthesis
Light phase of Photosynthesis |
Dark phase of Photosynthesis |
---|---|
It is also known as a photochemical phase. |
It is also known as a biochemical phase. |
It occurs only in the presenec of light. |
It can occur in the absence or presence of sunlight. |
Oxygen is liberated. |
CO2 is absorbed. |
ATP is produced. |
Glucose is produced. |
NADP is reduced. |
Reduced NADP is oxidized. |
Photolysis of water takes place. |
Photolysis of water does not occur. |
This phase or reaction occurs within the grana of the chloroplasts. |
This phase or reaction occurs within the stroma of the chloroplasts. |
Difference between Photosystem I and Photosystem II
PS I(Photosystem-I) |
PS II(Photosystem-II) |
---|---|
PS I is located on the outer surface of the thylakoid. |
PS II is located on the inner surface of the thylakoid. |
In this system, no molecular oxygen is evolved. |
Molecular oxygen is evolved as a result of the breakdown of water. |
A strong reductant is produced in this system, which causes a reduction of NADP+ to NADPH + H+. |
PSII supplies the electron, which is essential for PSI at the time of reduction of NADP+. |
This system takes part in both cyclic and non-cyclic photophosphorylation. |
It takes part only in PSI during the reduction of NADP+. |
PSI consists of pigment absorbing at longer wavelengths of light, which is 700 nm (p700). It also contains carotenoids and a single molecule of chlorophyll-a 700 (p700). |
PSII consists of pigment absorbing at shorter wavelengths of light, which is 680 nm (p680). |
The system comprises of chlorophyll-b, chlorophyll-a 670, chlorophyll a680, and chlorophyll-a 695. |
The system comprises of phycobilins, xanthophylls, chlorophyll-b, chlorophyll-a660, chlorophyll-a 670, and a single molecule of chlorophyll-a 680 (p680). |
PSI is active both in red and far-red light. |
PSII is active in far-red light. |
P700 is the photo center of this system. |
P680 is the photo center of this system. |
In this system, photolysis does not occur. |
In this system, photolysis takes place. |
The ratio of the chlorophyll carotenoid pigments 20-30 :1. |
The ratio of the chlorophyll carotenoid pigments 3-7 :1. |
It has an iron-sulfur(FeS) type reaction center. |
It has a quinone type (Q-type) reaction center. |
Concluding Remarks
Green plants and certain different life forms change light energy into chemical energy through the process of photosynthesis. They capture light energy and convert H2O, CO2, and mineral into oxygen and energy-rich organic compounds during photosynthesis. Oxygen is discharged into the earth through photosynthesis, which is essential for creatures and numerous microorganisms for the process of respiration. Photosynthesis also offers nourishment for a large portion of the living things on the earth. Consequently, it is called ‘pioneer’ of all processes, both abiological and biological.